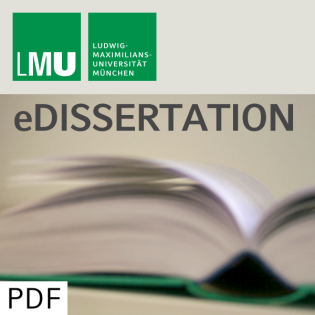
Imaging development and plasticity in the mouse visual system
Beschreibung
vor 17 Jahren
Neuronal activity, both intrinsically generated and sensory-evoked,
is known to play an important role in the development of the brain.
Sensory experiences continue to exert a strong influence on the
functional connectivity of neuronal circuits, especially in the
cerebral cortex, allowing for learning and adaptation to an ever
changing environment. The visual system provides a convenient and
well established model to study both development and
experience-dependent plasticity of neuronal circuits. The aim of
this thesis is to employ the mouse visual system to explore how
neuronal activity influences the formation of brain circuits and
mediates their experience-dependent modification later in life. In
the first part of this thesis (Chapter 2), I examined the role of
retinal activity in the formation of topographic maps in a target
region of retinal ganglion cells. It is generally assumed that in
order to obtain such highly precise and ordered maps during
development, spontaneous patterns of neuronal activity are crucial
for the refinement of connections. Applying intrinsic signal
imaging to mouse superior colliculus (SC), I confirmed this
assumption by showing that functional connectivity is less precise
in transgenic mice with disrupted patterns of retinal ganglion cell
activity. In comparison to normal mice, visual stimuli activated
larger, less defined regions in the SC in mice lacking early
retinal waves. Surprisingly, I also found that the overall
topographic organization was affected by the lack of correlated
spiking in the retina. Although the rough retinotopic organization
was maintained, the map showed substantial distortion, indicating
that patterned retinal activity before eye-opening plays a more
important role in topographic map formation than previously
thought. Later in development, sensory-evoked activity is equally
influential in shaping functional connectivity, since altered
sensory input induces strong changes in cortical circuitry. Closure
of one eye for a few days (monocular deprivation, MD), for
instance, substantially changes cortical responsiveness to the two
eyes, shifting ocular dominance (OD) towards the non-deprived eye.
This paradigm therefore provides a powerful model system for
experience-dependent plasticity. In Chapter 3, I used intrinsic
signal imaging to assess the magnitude of cortical responses evoked
by stimulation of the two eyes in order to explore OD plasticity in
mouse visual cortex. I confirmed recent, debated findings in
demonstrating strong MD-induced plasticity in adult animals, which
was mediated by partly different mechanisms than in juvenile mice.
I also found that restoring binocular vision after MD led to full
recovery of eye-specific responses at all ages. Interestingly, the
prior experience of altered sensory input seemed to be somehow
preserved in cortical circuits, such that subsequent cortical
adaptation to the same experience was improved. A second MD
resulted in much faster and more persistent OD shifts. This
enhancement of plasticity was highly specific, as it was only
observed for repeated deprivation of the same eye, indicating that
a lasting trace was established in cortical connections by the
initial experience. In Chapter 4, I explored OD plasticity in
greater detail by monitoring network activity at the level of
individual neurons with in vivo two-photon imaging of calcium
signals. Monitoring calcium transients associated with neuronal
activity in up to hundred cells simultaneously, enabled me to
examine MD-induced changes in the functional properties of each
neuron independently. I found that, in general, deprived eye
responses were weakened and non-deprived eye responses strengthened
after MD in juvenile mice, as was expected from previous population
response measurements. Neurons still dominated by deprived-eye
inputs, however, did not lose their responsiveness, but rather
exhibited enhanced responses following MD. This strongly suggests
that homeostatic plasticity acted on these cells during deprivation
and caused an up-scaling of their responsiveness, while neurons
also receiving substantial input from the non-deprived eye shifted
their responsiveness towards that eye. Both competitive and
homeostatic processes therefore seem to operate during OD
plasticity, depending on the distribution of functional inputs in
individual cells. In conclusion, the work presented in this thesis
provides further insight into the role of activity-dependent
mechanisms in determining and shaping functional connectivity in
the brain.
is known to play an important role in the development of the brain.
Sensory experiences continue to exert a strong influence on the
functional connectivity of neuronal circuits, especially in the
cerebral cortex, allowing for learning and adaptation to an ever
changing environment. The visual system provides a convenient and
well established model to study both development and
experience-dependent plasticity of neuronal circuits. The aim of
this thesis is to employ the mouse visual system to explore how
neuronal activity influences the formation of brain circuits and
mediates their experience-dependent modification later in life. In
the first part of this thesis (Chapter 2), I examined the role of
retinal activity in the formation of topographic maps in a target
region of retinal ganglion cells. It is generally assumed that in
order to obtain such highly precise and ordered maps during
development, spontaneous patterns of neuronal activity are crucial
for the refinement of connections. Applying intrinsic signal
imaging to mouse superior colliculus (SC), I confirmed this
assumption by showing that functional connectivity is less precise
in transgenic mice with disrupted patterns of retinal ganglion cell
activity. In comparison to normal mice, visual stimuli activated
larger, less defined regions in the SC in mice lacking early
retinal waves. Surprisingly, I also found that the overall
topographic organization was affected by the lack of correlated
spiking in the retina. Although the rough retinotopic organization
was maintained, the map showed substantial distortion, indicating
that patterned retinal activity before eye-opening plays a more
important role in topographic map formation than previously
thought. Later in development, sensory-evoked activity is equally
influential in shaping functional connectivity, since altered
sensory input induces strong changes in cortical circuitry. Closure
of one eye for a few days (monocular deprivation, MD), for
instance, substantially changes cortical responsiveness to the two
eyes, shifting ocular dominance (OD) towards the non-deprived eye.
This paradigm therefore provides a powerful model system for
experience-dependent plasticity. In Chapter 3, I used intrinsic
signal imaging to assess the magnitude of cortical responses evoked
by stimulation of the two eyes in order to explore OD plasticity in
mouse visual cortex. I confirmed recent, debated findings in
demonstrating strong MD-induced plasticity in adult animals, which
was mediated by partly different mechanisms than in juvenile mice.
I also found that restoring binocular vision after MD led to full
recovery of eye-specific responses at all ages. Interestingly, the
prior experience of altered sensory input seemed to be somehow
preserved in cortical circuits, such that subsequent cortical
adaptation to the same experience was improved. A second MD
resulted in much faster and more persistent OD shifts. This
enhancement of plasticity was highly specific, as it was only
observed for repeated deprivation of the same eye, indicating that
a lasting trace was established in cortical connections by the
initial experience. In Chapter 4, I explored OD plasticity in
greater detail by monitoring network activity at the level of
individual neurons with in vivo two-photon imaging of calcium
signals. Monitoring calcium transients associated with neuronal
activity in up to hundred cells simultaneously, enabled me to
examine MD-induced changes in the functional properties of each
neuron independently. I found that, in general, deprived eye
responses were weakened and non-deprived eye responses strengthened
after MD in juvenile mice, as was expected from previous population
response measurements. Neurons still dominated by deprived-eye
inputs, however, did not lose their responsiveness, but rather
exhibited enhanced responses following MD. This strongly suggests
that homeostatic plasticity acted on these cells during deprivation
and caused an up-scaling of their responsiveness, while neurons
also receiving substantial input from the non-deprived eye shifted
their responsiveness towards that eye. Both competitive and
homeostatic processes therefore seem to operate during OD
plasticity, depending on the distribution of functional inputs in
individual cells. In conclusion, the work presented in this thesis
provides further insight into the role of activity-dependent
mechanisms in determining and shaping functional connectivity in
the brain.
Weitere Episoden


vor 16 Jahren
Kommentare (0)